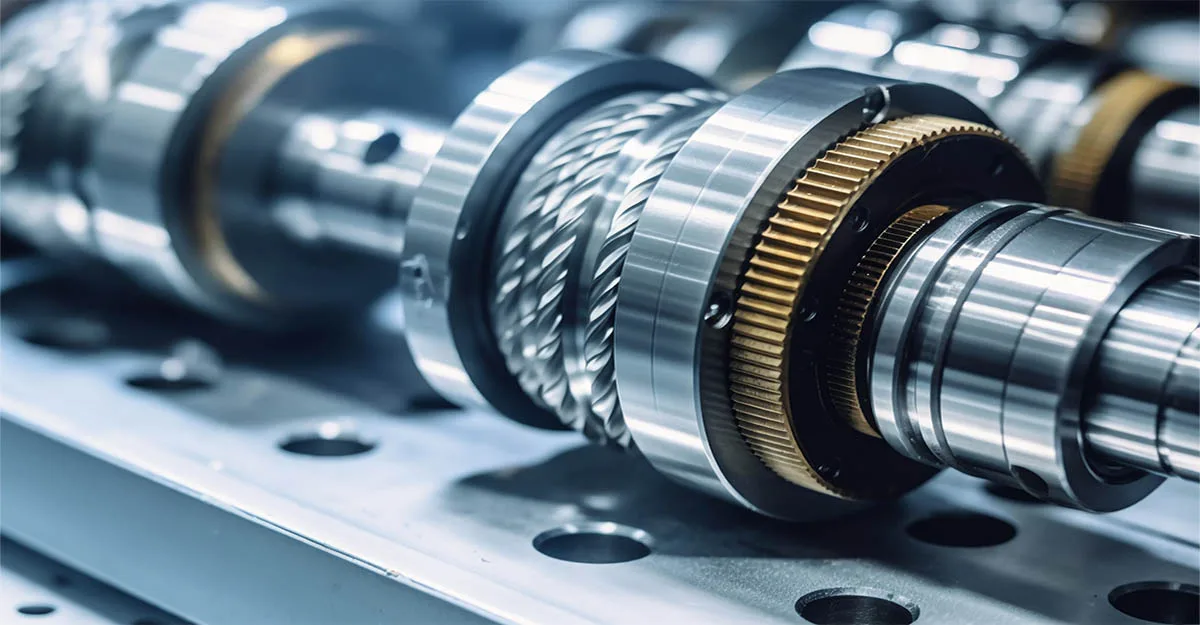
We often talk about the castability, forgeability, machinability, weldability, and heat treatment of stainless steel—but do we really understand what these terms mean? Many people may have a rough idea of how a certain process works, but when asked to explain it clearly, they might fall short. This article summarizes the most common stainless steel processing methods as a reference for both technical and industrial use.
Stainless Steel: Why It’s Widely Used
Stainless steel is known for its excellent corrosion resistance, which is why it is widely used across various industries. There are numerous grades of stainless steel available today, each differing in formability, strength, and workability. Among them, 304 stainless steel and 316 stainless steel are the most common.
304 (A2 stainless steel): Contains 18–20% chromium and 8–10% nickel.
316 (A4 stainless steel): Contains about 16% chromium, 10% nickel, and 2–3% molybdenum.
The key difference is that 316 contains molybdenum, which enhances resistance to chloride corrosion. This makes 316 particularly suitable for chemical resistance in high-temperature environments, such as resistance to fatty acids and sulfuric acid. It can withstand temperatures up to 871°C, making it ideal for use in marine applications.
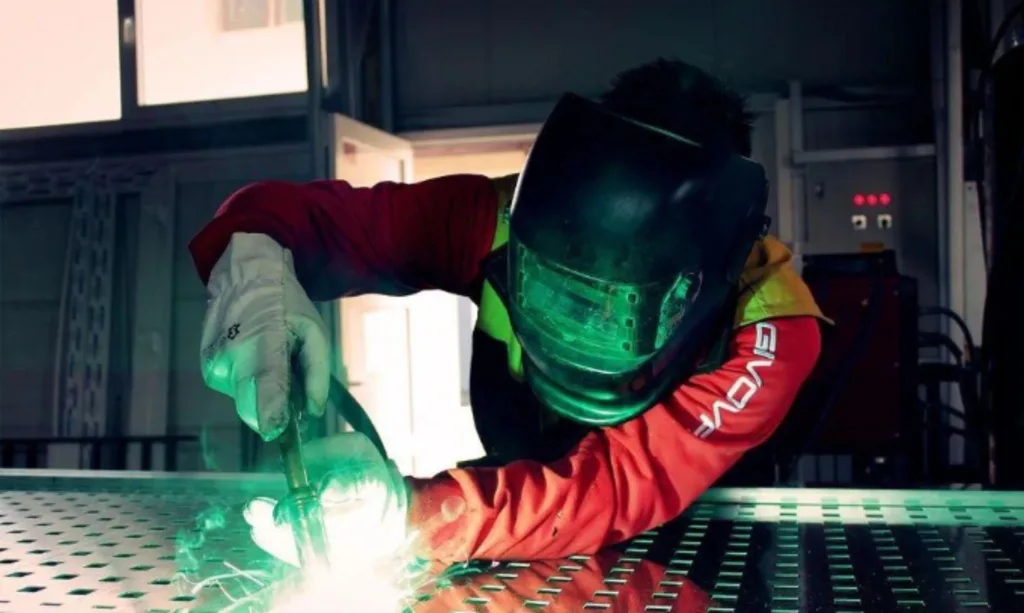
Machinability
304 stainless steel is easier to machine than 316.
It is also easier to clean and is often used for decorative finishes.
316 stainless steel, by contrast, is more difficult to cut and requires special tools. It is typically used only when other materials fail to meet the application’s requirements.
Machining Tools & Techniques
Both 304 and 316 require heavy-duty equipment due to significant vibration during processing.
Carbide or high-speed steel (HSS) tools are recommended, with HSS performing better at lower cutting speeds.
Work hardening is common across all 300-series steels, with 316 being particularly prone. To counteract this:
Use sharp tools.
Operate at lower speeds and higher feed rates.
For complex parts, use deep cuts and high feed rates to reduce work hardening.
Castability of Stainless Steel
Castability refers to how well stainless steel can be cast into sound components, involving three key properties:
Fluidity: The ability of molten metal to fill the mold.
Shrinkage: The degree to which metal volume contracts upon solidification.
Segregation: Uneven distribution of chemical composition during solidification.
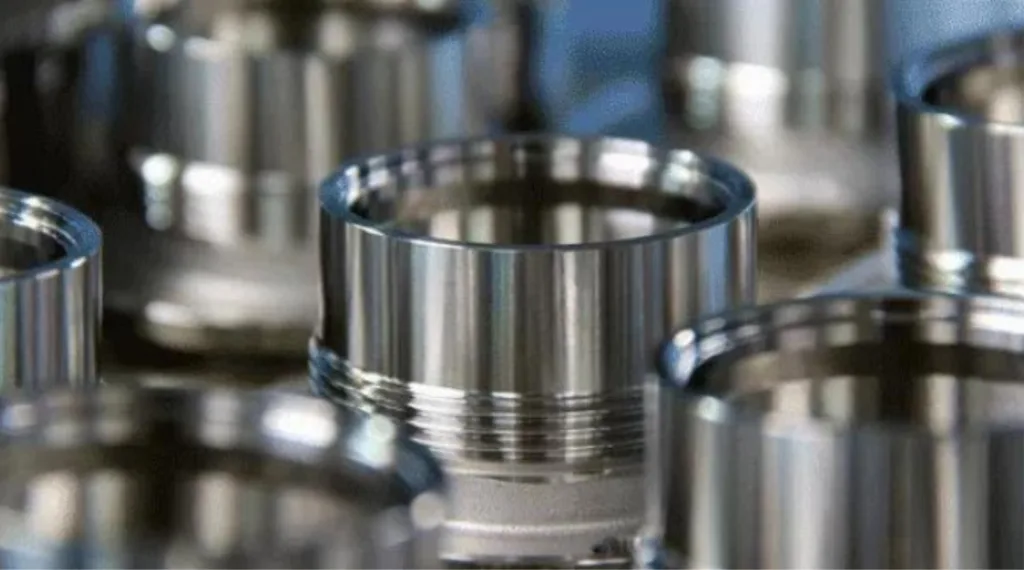
Tips for Cutting Stainless Steel
For cutting bars with a diameter ≤ 40 mm:
Use high-speed steel cutting tools for good results.
For larger diameters:
Use carbide tools to maintain efficiency at low cutting speeds.
Important cutting tips:
Keep the tool’s rake angle at 0°. Stainless steel generates significant friction and chip compaction, which may cause tool breakage if not properly managed.
Avoid large tool nose radii, which can reduce rear relief angles and increase side wear.
Regrind tools regularly, especially the side relief face. Excessive wear narrows chip grooves and causes tool failure.
Polish the tool face to reduce adhesion wear and improve tool life.
Apply a negative chamfer (~0.2mm) at the cutting edge to prevent chipping and increase edge durability.
Recommended carbide grade: YG8
Cutting speed: Approx. 60 m/min
For diameters 40–80 mm, a single-pass cut is achievable.
Use safety precautions to break long chips and avoid injury.
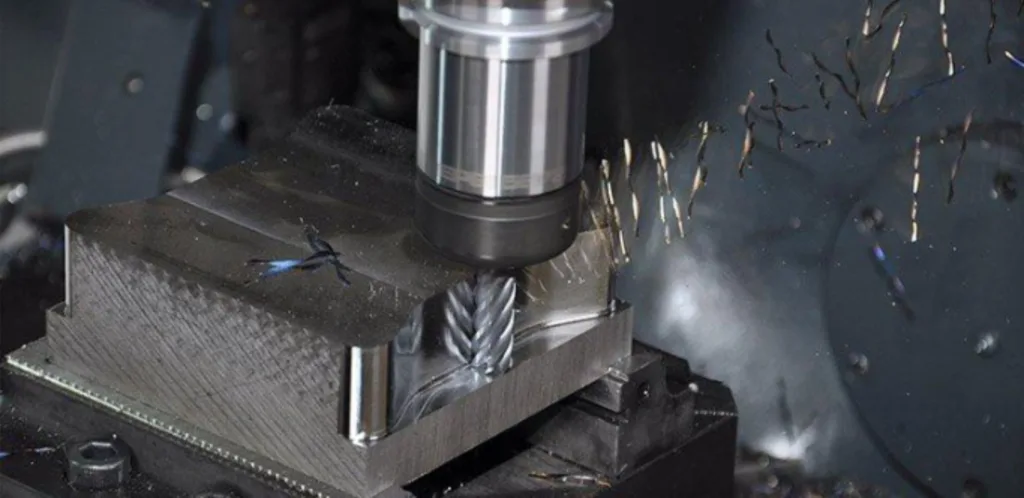
Forgeability
Forgeability refers to stainless steel’s ability to undergo deformation under pressure (hot or cold) without cracking. This includes hammer forging, rolling, drawing, and extrusion. Chemical composition plays a crucial role in determining forgeability.
Weldability
Weldability describes stainless steel’s adaptability to welding processes, including:
Joint Integrity: Susceptibility to defects under specific welding conditions.
Performance Suitability: Ability to meet service requirements after welding.
Heat Treatment Processes
1. Annealing
Involves heating the steel to a certain temperature, holding it for a time, then cooling slowly.
Purpose: Reduce hardness, relieve stress, improve ductility, and prepare for further processing.
2. Normalizing
Steel is heated 30–50°C above its upper critical point and cooled in air.
Purpose: Improve strength, refine grain size, eliminate structural defects, and prepare for further heat treatment.
3. Quenching
Steel is heated above its critical point and rapidly cooled to form a martensitic structure.
Purpose: Increase hardness, strength, and wear resistance. Common methods include salt bath, martensitic step, bainitic, surface, and localized quenching.
4. Tempering
After quenching, steel is reheated below the lower critical point, held, and cooled.
Purpose: Reduce internal stresses, improve toughness and ductility while retaining hardness.
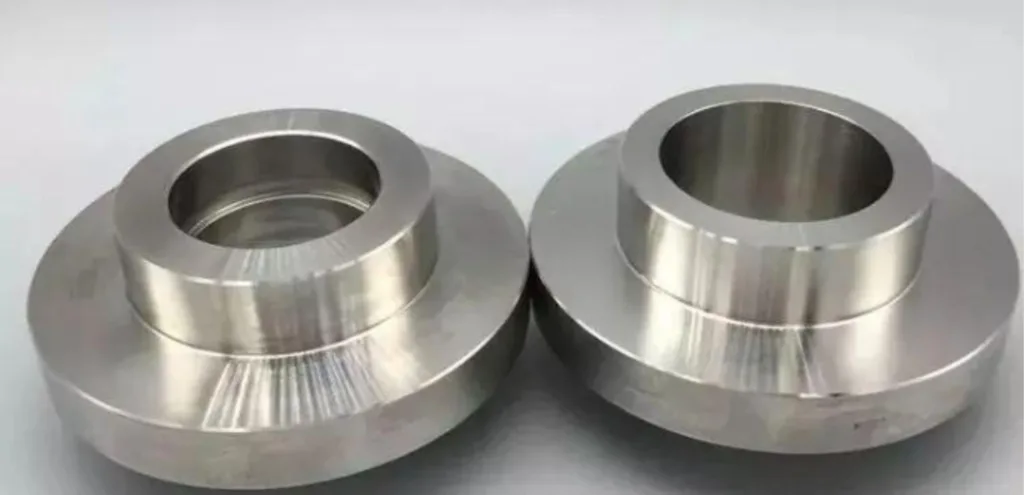
5. Solution Treatment
Involves heating to a high-temperature single-phase zone, holding, then rapidly cooling.
Purpose: Create supersaturated solid solutions and enhance overall performance.
6. Precipitation Hardening
Occurs when solute atoms or particles precipitate from a supersaturated solid solution, strengthening the material.
Example: Austenitic stainless steel undergoes this after solution treatment or cold working.
7. Aging Treatment
Material is stored at room or elevated temperature after solution treatment or plastic deformation.
Purpose: Relieve internal stress, stabilize structure and dimensions, and improve mechanical properties.
Hardenability
Hardenability describes how deep and uniformly a material can harden during quenching. It is affected by:
Chemical composition (especially alloying elements)
Grain size
Heating temperature and holding time
High hardenability means better mechanical performance across the section, lower distortion, and reduced cracking risk.